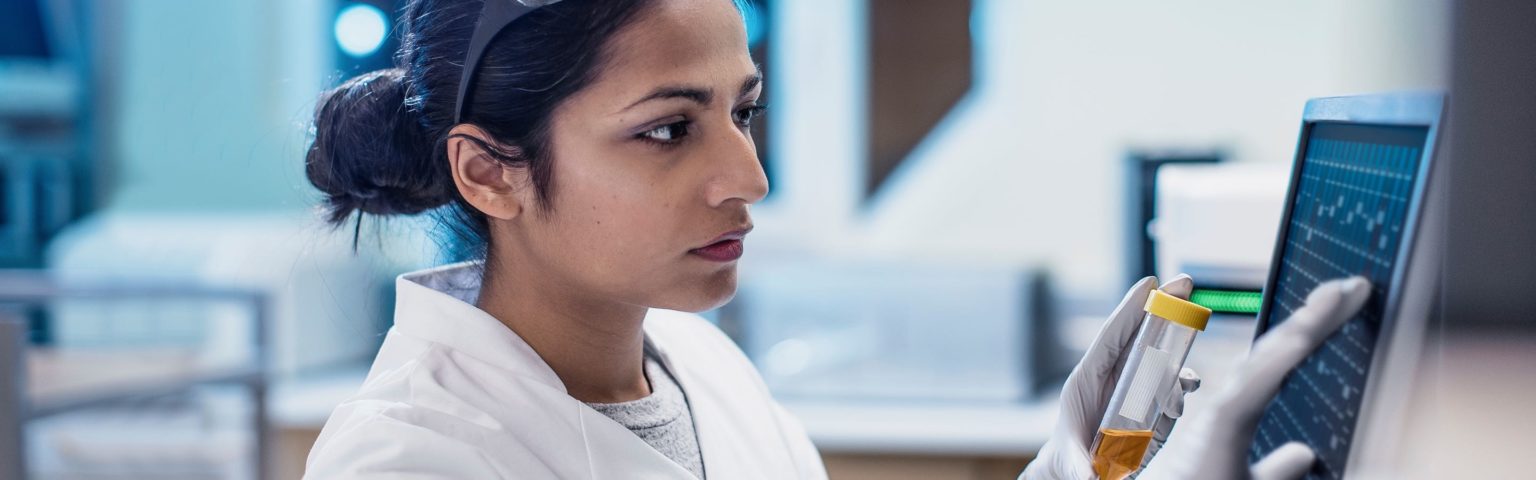
Mike Gauthier
09.05.2019
The What, Why and How of Getting Valuable Reliability Insights
We’re going to give you the benefit of the doubt that you’re already doing oil analysis on the lubricants serving your critical rotating equipment.
Most plants do oil analysis at least in some form from time to time, recognizing that machinery lubricant performs a crucial role in reliability. That is, to create and maintain a film between internal surfaces that reduces friction, heat and wear.
The fundamental purpose of oil analysis is to make sure the oil is capable of doing this important job. But you can learn a lot more from oil analysis than whether your lubricant is any good – if you get some real experts on your side, you do a comprehensive oil analysis program and you stick with it.
Here’s an overview of what your lab is looking for, why they’re looking for it, and how they’ll find they answers.
What they’re looking for: Glaring problems.
Why: If something seems amiss at the first pass, it probably needs immediate attention.
How: Visual/crackle inspection.
This step is kind of like triage for oil analysis – trying to categorize any obvious or potential problems and help guide subsequent tests. It starts with visual observation, looking for discoloration, water separation or flaking of large particles.
There’s a smell test, too. The lab tech wafts the sample, sniffing for acids, fuels or cleaning agents that could suggest either an unwanted chemical reaction or contamination in the oil.
The process then moves to the “crackle” test to look for significant water content or other undesired liquids in the oil. This test involves heating the sample on a hot plate to 325-400 °C. If there’s water in the sample, it will start to make a crackling sound. If it bubbles, but doesn’t crackle, that’s a good indication there’s some kind of refrigerant in there. Either one could cause trouble, so it’s time for more precise testing.
In situations where the lubricating film layer is only 1 micron thick, you could imagine that any contaminants that are present can create major damage, so we try to eliminate as many as possible.
What they’re looking for: Water.
Why: Water dilutes the lubricating film of your machinery lubricant, breaks down important additives and can cause corrosion of equipment components.
How to look for it: Karl Fischer titration, Fourier Transform Infrared (FTIR) Spectroscopy.
In heavy-duty rotating equipment, free and emulsified water can be vaporized, which can lead to significant wear in the machine. Water can also accelerate oxidation that breaks down the oil. In synthetics, water can cause formation of destructive acids.
Suffice it to say, you don’t want water in your equipment lubricant!
If a crackle test shows the presence of water, Karl Fischer is the typical next step. It uses a reagent that reacts with water to measure the total presence up to 1% (10,000 ppm) of free, dissolved and emulsified water in an oil sample.
Water can also be identified, among other contents of your oil, through an advanced method called Fourier Transform Infrared spectroscopy, or FTIR.
What they’re looking for: Viscosity.
Why: It’s the fundamental characteristic that determines your oil’s ability to do its job.
How: With the lubricant bathed in water in a tube heated to 40 °C, the typical viscosity test measures the time the oil takes to flow between two points.
Viscosity that’s too high means the lubricant may not flow well enough to reduce friction, which may allow greater wear in the machine, speed up oxidation, advance sludge formation and necessitate greater power consumption to overcome increasing heat.
Viscosity that’s too low, on the other hand, means your oil can’t separate enough to protect the components. This condition can lead to many of the same problems as with high viscosity.
What they’re looking for: Wear particles and other contaminants.
Why: Metals in your oil are a sign that internal components are being worn away.
How: Elemental analysis with inductively coupled plasma (ICP); direct read (DR) ferrography; wear particle analysis (WPA) via analytical ferrography.
Every sample should be checked for concentrations of iron and tin, aluminum, silicon, boron, phosphorus or titanium. Titanium in particular means serious trouble because it’s a very hard metal, hinting that softer surface metals on your component have already been worn away.
In an ICP test, the oil sample is introduced to a plasma flame arc. The resulting light emission reveals 21 key elements common to oil and contaminants and additives. In addition to wear particles, it can help identify the presence of calcium, which could be from a detergent or a water source. Detergents can be a necessary additive, but water, as we said before, is not good.
A DR analysis looks for concentrations of larger, ferrous (iron-containing) particles. It involves flowing a sample over a magnet that’s increasing in strength. The presence of these larger particles raises concerns about shaft debris and other significant wear issues.
WPA ferrography is usually done as a secondary test after wear particles are uncovered. It involves making a slide on which the particles are lined up over a magnet. The behavior of the particles reveals a variety of nonferrous metals that are known to experienced analysts to be contaminants resulting from wearing down of gears, driveshafts, cylinders, etc. Seasoned analysts can even identify the type of wear that’s occurring – spalling, shearing, etc.
FTIR is another additional step that helps reveal a wide range of contaminants and problems. Experts in this highly specialized protocol can identify, analyze and interpret the implications of oxidation, hydroxides, alcohols, nitrates, soot and various acids in your oil.
What they’re looking for: Particulate – dust and dirt.
Why: Dirty oil is ineffective oil.
How: LaserNet Fines particle analysis.
About 80% of bearing failures can be directly attributed to lubrication problems including particulate contamination.
The LaserNet Fines process involves directing a laser through a flowing sample of oil and examining the shadows created by particles blocking the laser. The sample is vibrated via an ultrasonic bath to produce air bubbles that can then reveal much smaller particles.
What they’re looking for: Additives.
Why: Additives impart key properties on equipment lubricants for the specific needs of an application.
How: Elemental analysis with inductively coupled plasma (ICP)
Dispersants help protect components against abrasion from wear products by enveloping particles and suspending them in the oil so that they may be easily flushed and removed from the system.
Anti-wear (AW) and extreme pressure (EP) additives react with a component’s surfaces to form a thin protective layer to prevent metal-to-metal contact. Silicon, although it’s often found in just plain old dirt, is sometimes a valid defoamant additive.
There’s a specified concentration at which each of these additives is most effective. A sudden change in the concentration may mean that the lubricant has been contaminated with a different lubricant or additive. In that case the additive you need won’t do the job it’s supposed to do.
ICP elemental analysis helps identify and analyze the presence and concentration of these and other additives to ensure your lubricant is up to the job.
What they’re looking for: Acid.
Why: Rising acidity suggests a breakdown in the chemical composition of the lubricant. Decreasing acidity tells you there’s a chemical reaction.
How: Total acid number (TAN)
The acid number is expected to increase over the lifetime of the lubricant as a function of lubricant aging. At a certain point, it will start to corrode the metals in the equipment. That’s why it’s important to monitor.
Certain situations are going to make acids rise and drop. Synthetic lubricants break down under different conditions. Acid production can also be a reaction to some kind of catalyst, like water. It’s related to the level of oxidation of the oil – the higher the oxidation, the higher the acid number.
The breadown of some additives can also cause your acid number to decrease. As your additives deplete over time, the acid number will drop until it hits a minimum, then rise. It rises at that point because there’s now genuine acidic material in your oil. This acid buildup in oil is a bad thing.
The TAN test involves introducing a solution that reacts with acid and measures the electrical discharge that occurs due to the chemical reaction between the substances.
For engine oils, labs typically do the reverse of this test, calculating the total base number (TBN). You want the oil base number to be high and acid numbers to be steady because the engine is continuously producing acid. And a high base number indicates that the lubricant is capable of neutralizing – or chemically eliminating – the acid.
It Isn’t Just About These Tests
Effective oil analysis takes more than technical expertise in these testing processes. Experience with the specific mechanical applications, the characteristics of industrial environments and the common behaviors in these facilities that impact the condition of the oil are all also vital.
Combine that depth of expertise with a thorough oil analysis program that includes frequent sampling (quarterly at a minimum); application data regarding the machine, its operating environment, its workload, etc.; and sampling from multiple points in your process and your facility to look for root causes outside the machine.
We call this more complete application of knowledge Oil Intelligence. That’s how you uncover trends and anticipate and prevent failures.
- Category:
- Oil Analysis
- Contamination